Learning from Nature: The Future of Design
Learning from Nature: The Future of Design demonstrated how designers are finding sustainable solutions to human challenges by emulating nature’s time-tested patterns and strategies. The exhibition invited all visitors to get involved in learning about biomimicry and designing based on ideas drawn from nature. The exhibition originated in March 2020 at Museum of Design Atlanta (MODA). An adapted version of it was at the Kendeda Building from 2021 to 2023.
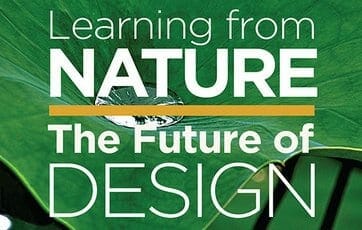
Introduction
Biomimicry is about valuing nature for what we can learn, not what we can extract, harvest, or domesticate. In the process, we learn about ourselves, our purpose, and our connection to each other and our home on earth. — Biomimicry Institute
Have a design or engineering problem? The answer may be found in the natural world! Life appeared on Earth about 3.8 million years ago. Since that time, nature’s nonstop process of research and development has allowed organisms to adapt to changing circumstances and needs in order to become more resilient, sustainable, and efficient. Biomimicry — the design strategy that is the subject of this exhibition — is the practice of learning from nature’s adaptations and emulating them to provide solutions to human challenges. It is innovation inspired by nature.
Biomimicry is reframing how we think about nature. This discipline asks us to observe nature closely, to investigate how the natural world works, and to apply our observations and learnings to the human world.
Engaging with biomimicry as a design strategy helps us understand that human activity takes place in the context of larger natural processes and cycles. It challenges us to learn from nature rather than simply using it for our benefit.
Biomimicry is especially important as we enter an era of unprecedented climate change that will challenge existing systems and ways of life. As this exhibition demonstrates, many designers and engineers who practice biomimicry are exploring ways to reduce our impact on natural ecosystems and to restore our symbiotic relationship with the world around us.
Speedo Fastskin
Sharkskin is made of tiny structures shaped like curved, grooved teeth that are called dermal denticles or placoid scales. The denticles decrease drag and turbulence, allowing the shark to swim faster and more quietly.
In the 1990s, Speedo’s Global R&D center, Aqualab, recognized that companies were not considering how water interacted with the smooth materials they were using to make swimsuits. Their interest in this issue inspired them to visit a natural history museum where they learned about shark denticles. Speedo then went on to develop Fastskin, a suit with shark-like denticles that channels water over a swimmer’s body 3% more efficiently than suits made of traditional materials.
Fastskin suits were first introduced at the 2000 Olympics in Sydney. At those games, 83% of the competitive swimming medals were won by swimmers wearing Fastskin and suit wearers broke 13 of 15 world records.
New technical suits from all brands with maximum body coverage were subsequently banned from competition in 2010 as a form of “techno-doping,” though there is some controversy as to whether it was the texture of the suits that made them faster.
Speedo’s discovery has impacted more than swimming. The denticles on sharks are inspiring organizations like NASA to develop materials that improve the efficiency of boats and aircraft, as well as reduce the energy they consume.
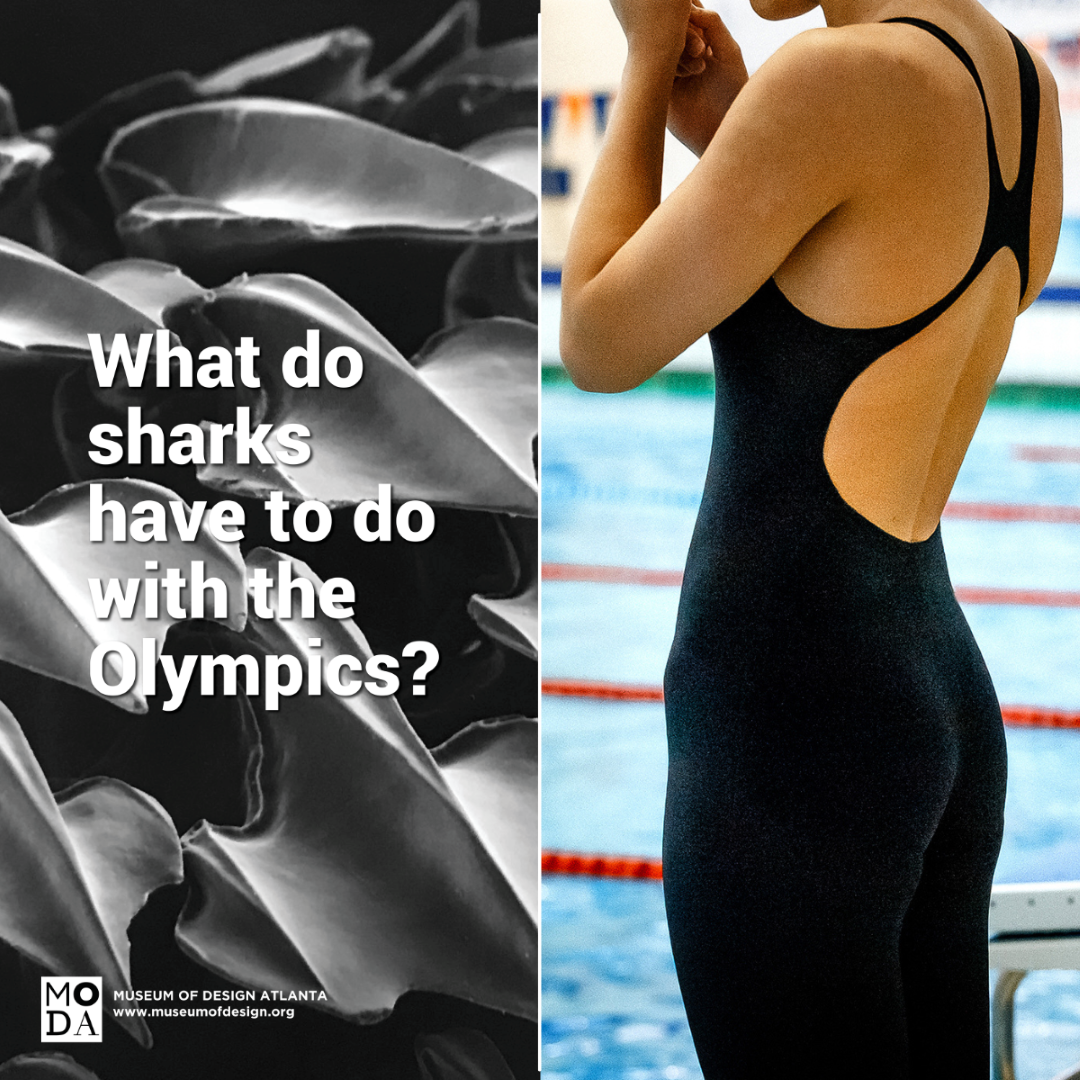
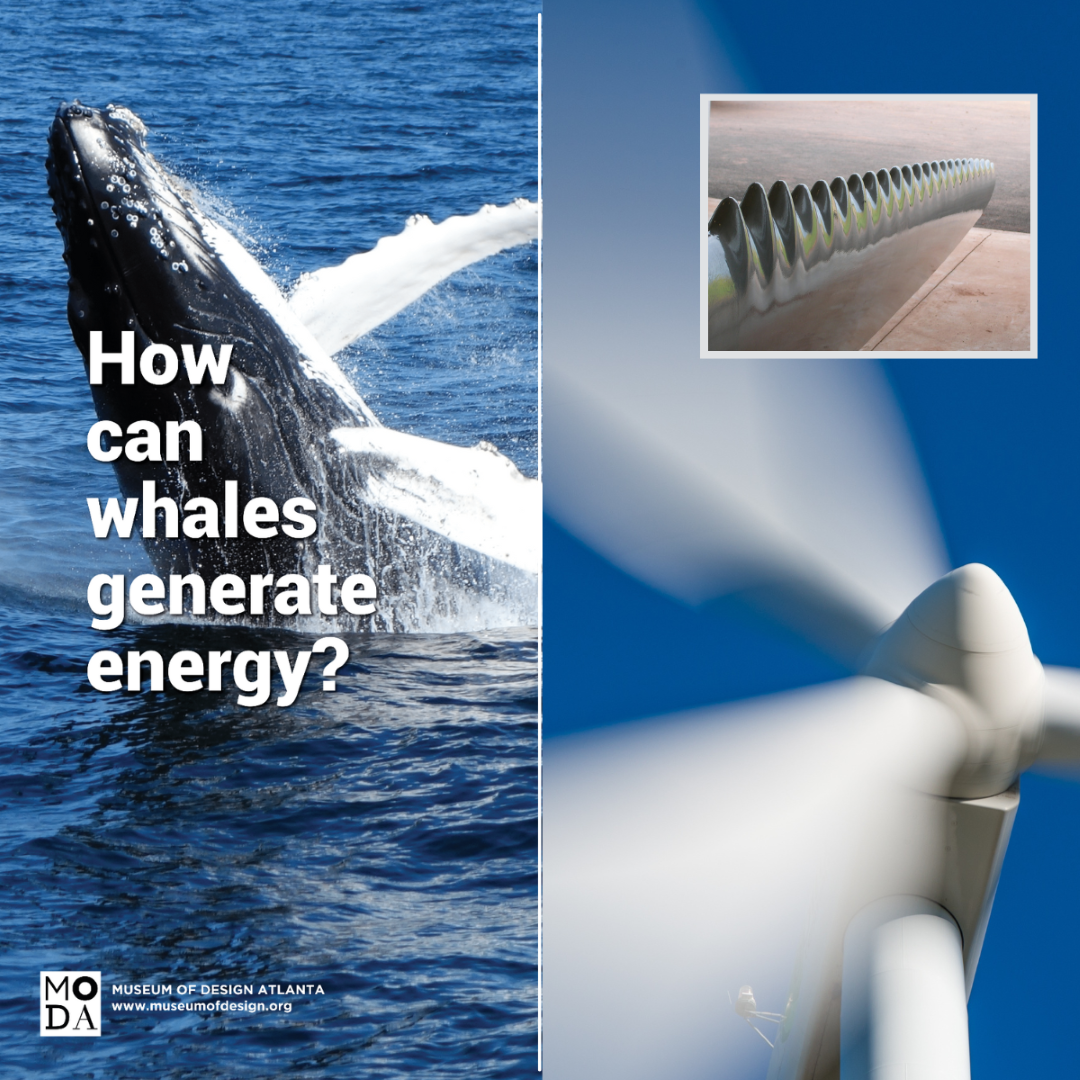
Tubercled Turbine Blade
Wind turbines operate on a simple principle. The energy in the wind turns two or three propeller-like blades around a rotor. The rotor is connected to the main shaft, which spins a generator to create electricity.
While wind power is a great alternative to fossil fuels, it has some limitations. Current wind turbine blades require steady, high winds to generate electricity. And they make a lot of noise.
With his partners in the company WhalePower, Frank E. Fish found a way to make air turbines more efficient when he noticed that the edge of humpback whale flippers have tubercles or bumps. The tubercles are key to how the whale maneuvers through water because they decrease drag and increase lift.
Fish applied this learning to turbine and fan blades in order to reduce noise and make them more efficient. Tests show that tubercled wind turbine blades produce 20% more power each year. They are also quieter and require lower wind speeds. This increases the amount of time they can be used and the number of locations in which they are a viable source of alternative energy.
ORNILUX Mikado
Between 36.5 and 98.8 million birds die each year in the United States when they collide with windows. Unlike humans, birds cannot read the architectural cues that indicate the presence of glass. Instead, the reflectivity of glass signals vegetation and sky to birds, while the transparency signals potential flight paths through buildings.
Researchers in the Arnold Glas Group found a solution to this problem by investigating why birds don’t fly through spider webs. They determined that spider webs contain ultraviolet (UV) reflective strands of silk. Because birds see in UV, the webs are visible to them.
The researchers emulated this natural strategy by applying a pattern of UV-reflecting coating to glass panels to develop ORNILUX glass. While birds can see the pattern on the glass and therefore avoid collisions, the pattern is faint or imperceptible to humans. ORNILUX glass is now widely marketed and independent field testing shows that 76% of the birds that approach an ORNILUX panel turn away from it.
Photos courtesy of Arnold Glas Group.
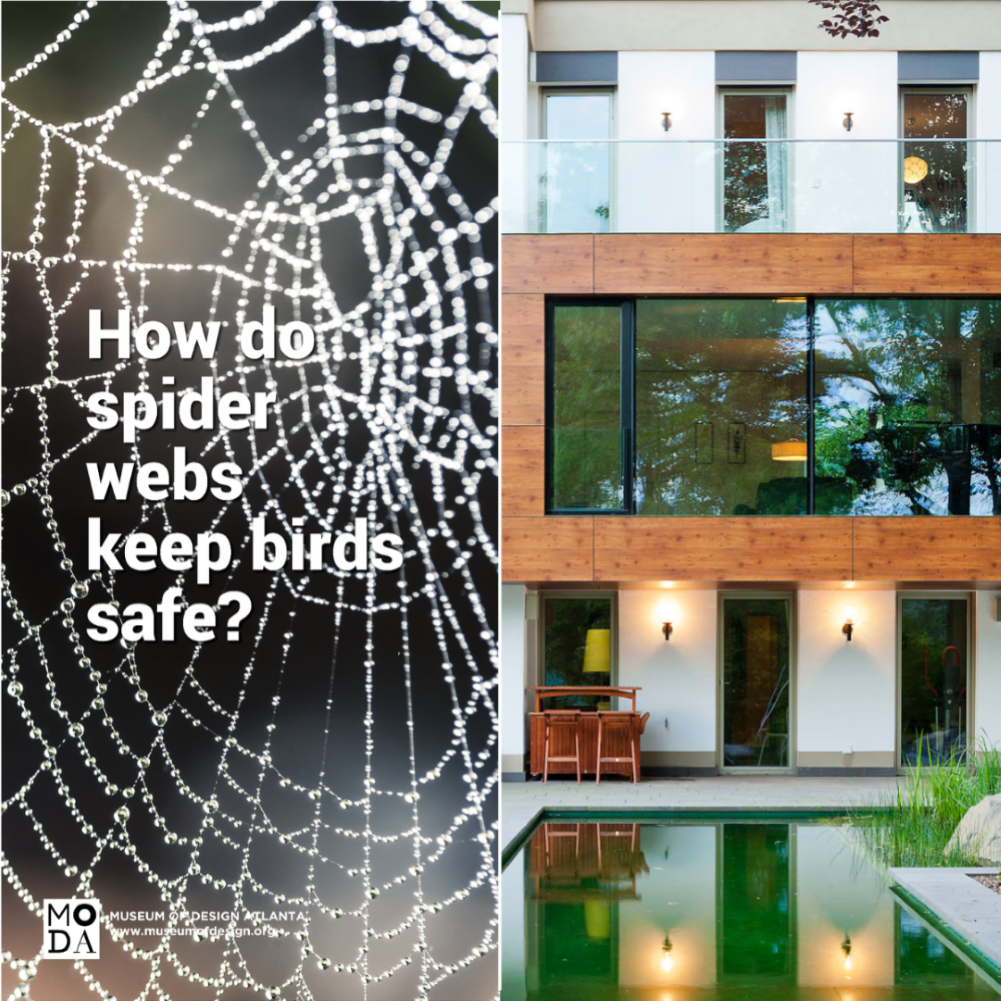
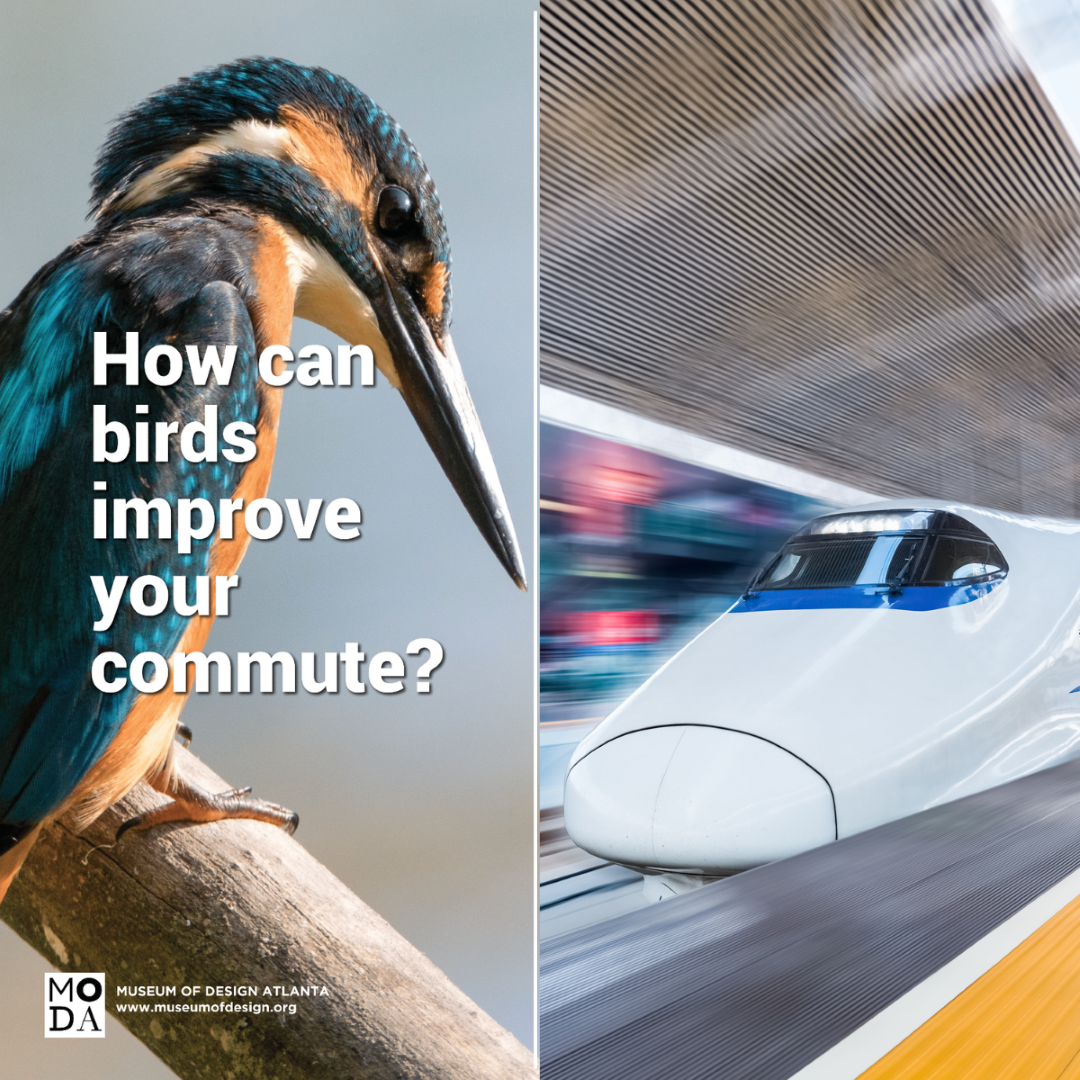
Shinkansen Bullet Train
In the 1990s, the Japanese bullet train system faced a problem. The 500 series Shinkansen trains — which can travel over 200 miles an hour — created sonic booms when they exited from tunnels. The sonic booms exceeded environmental noise standards and disturbed nearby residential areas.
Eiji Nakatsu, chief engineer of the bullet train at the time, happened to be an avid birdwatcher. Inspired by the kingfisher’s ability to dive into water without making a splash, he proposed modeling the front of the train front after the kingfisher’s beak.
The new train design was able to prevent sonic booms from occurring. It also allowed the train to move 10% faster with 15% less energy due to improved aerodynamics.
StoColor Lotusan
When water falls on a lotus leaf, it immediately forms droplets and rolls off the surface, picking up particles of dirt along the way. This self-cleaning property fascinated Wilhem Barthlott. While he was studying lotus leaves in 1977, he discovered that they have a nanoscopic architecture on their surface that prevents water droplets from adhering to them. This phenomenon, called “ultrahydrophobicity,” has been nicknamed “the lotus effect.”
Working with Sto Corp., a building materials company that has been championing sustainability since 1963, Barthlott helped to develop StoColor® Lotusan®, a wall coating for building exteriors that mimics the surface of a lotus leaf.
StoColor® Lotusan® is highly water-repellent. When applied to a wall, it forms microstructures that cause water to run off, helping keep building exteriors dry. The texture of the paint also helps keep buildings clean. Just as with a lotus leaf, water droplets that roll of Lotusan® pick up dirt. This reduces the need for re-painting and saves on maintenance costs and resources.
Photo courtesy of Sto Corp.
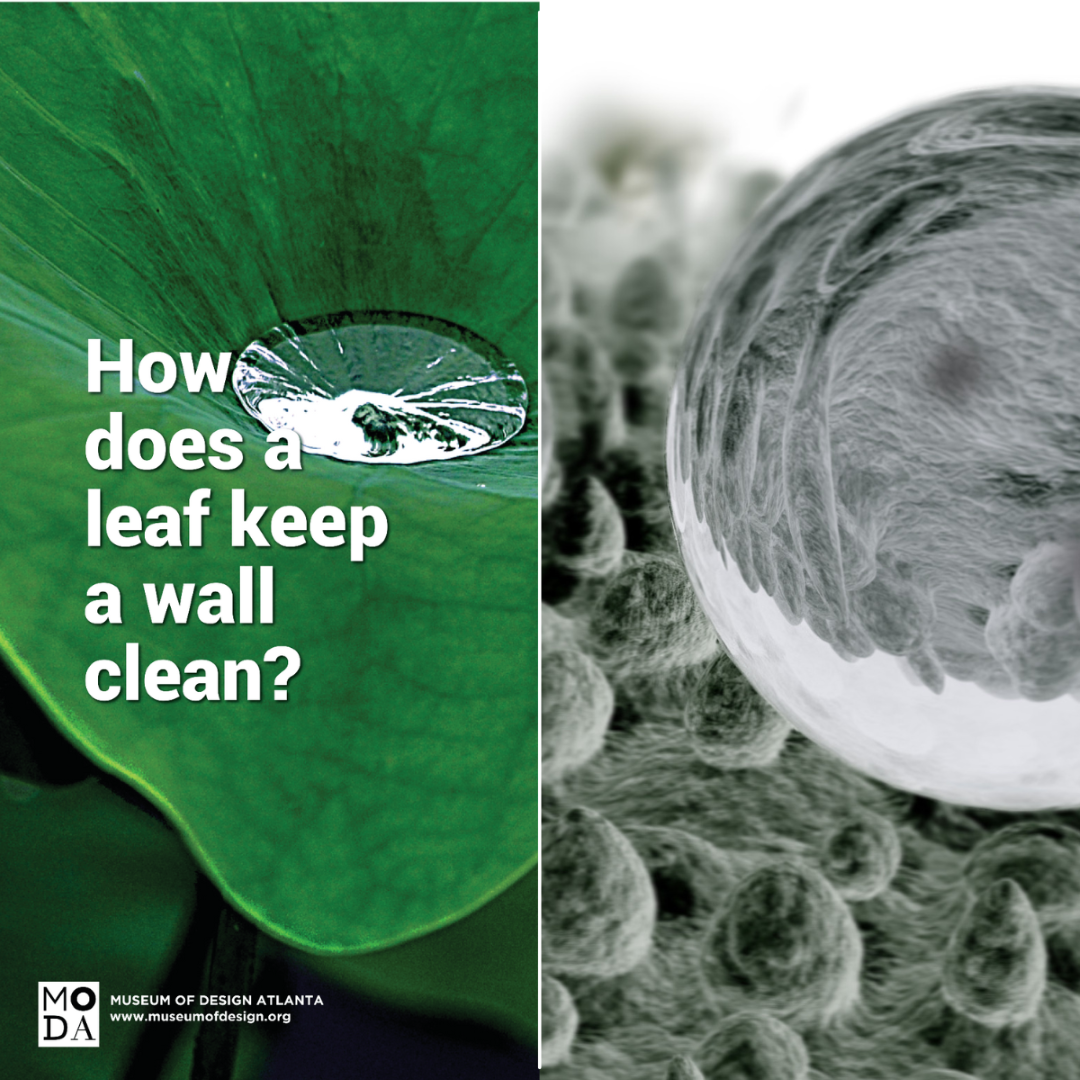
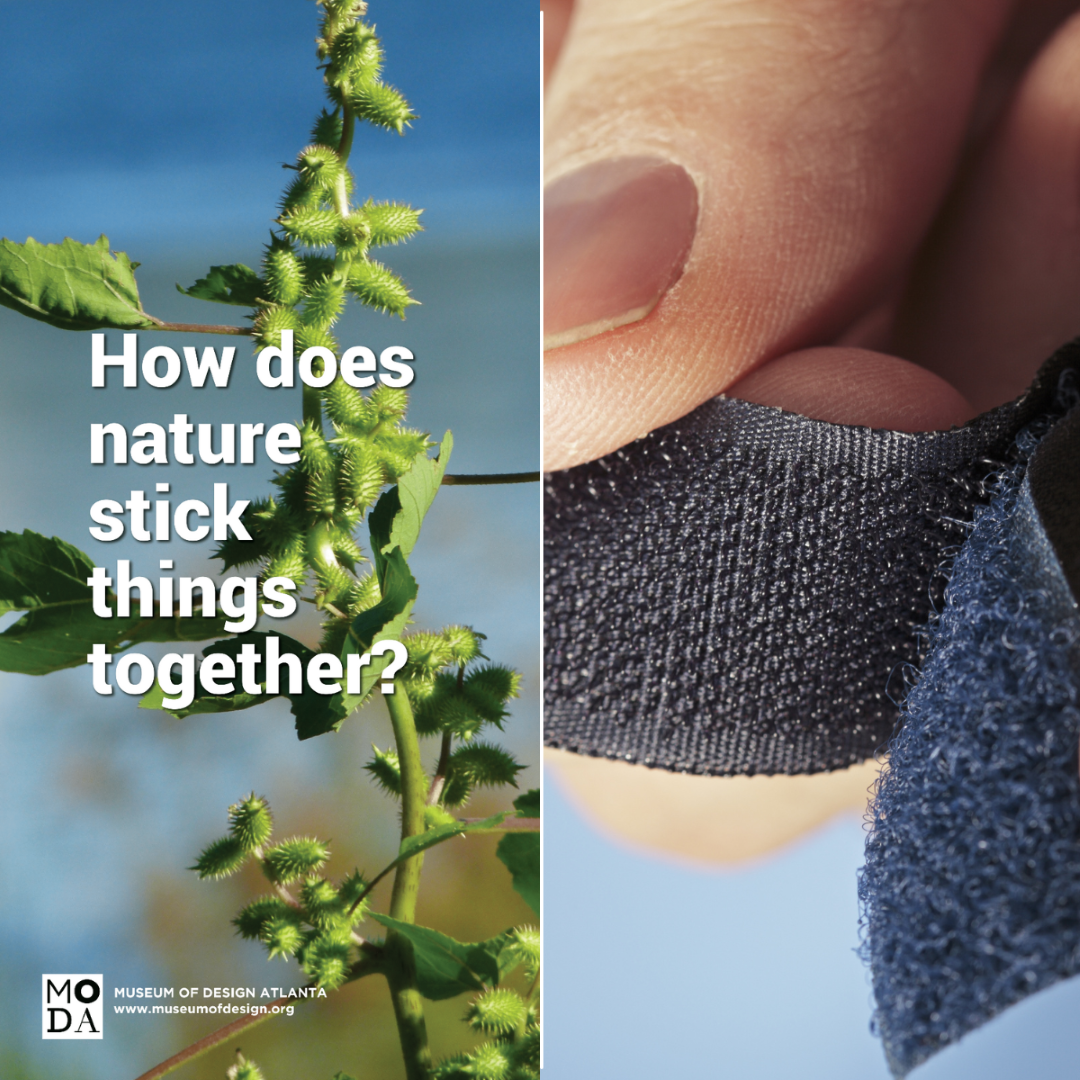
VELCRO®
After hunting in the woods, Swiss engineer George de Mestral noticed that cockle-burs (burdock seeds) were stuck on his pants and in the fur of his dog. Wondering how they attached themselves, he examined the seeds under a microscope and saw that they had tiny hooks that engaged with the loops in the fabric of his pants.
Understanding that a “hook and loop” fastener modeled on nature might be useful, de Mestral collaborated with friends in the weaving business to create VELCRO®, a fabric strip with tiny hooks that "mates" with another fabric strip with loops, to form a temporary attachment. They derived the brand name from the French word velour (“velvet”) and crochet (“hook”).
Introduced in 1960, VELCRO® was first adopted by the aerospace industry as an aid to getting in and out of bulky space suits. A few years later, manufacturers of children's clothing and sports apparel realized the possibilities.
Gecko Tape & Adhesives
For a long time, scientists were baffled by a gecko’s ability to “stick” to surfaces without the help of liquid or heat and without leaving residue behind.
In 2003, Andre Geim and fellow researchers discovered the millions of tiny hairs on gecko feet allow them to climb. The hairs are so fine that they can get very close to minute contours in walls and ceilings, which causes a physical bond called the van der Waals force. Electrons from gecko hair molecules interact with electrons from wall molecules to create an electromagnetic attraction that adheres the gecko to the surface. The adhesion is so strong that a single gecko foot can support about 2.25 pounds.
Now, Geim and other engineers have found a way to create adhesives that mimic this property. These materials have millions of tiny fibers, so they react like gecko feet when stuck on a surface. The products support weight, but they can also be easily removed and re-stuck in another location.
Geckos also inspired the adhesive squares that are keeping the carpet beneath your feet in place. In 2006, Interface introduced TacTiles, 3” adhesive squares that provide a way to stick their carpet squares together without gluing them to the floor.
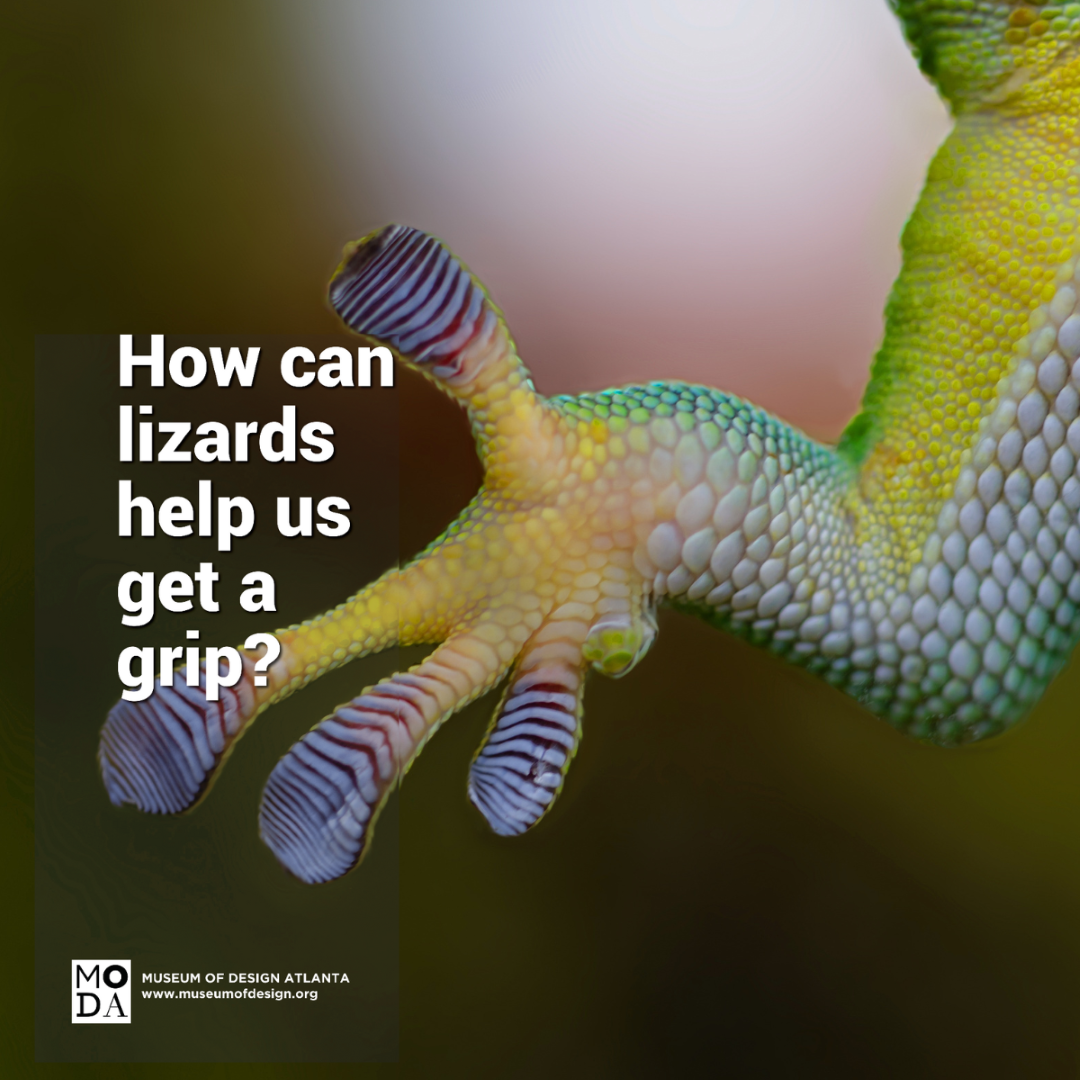
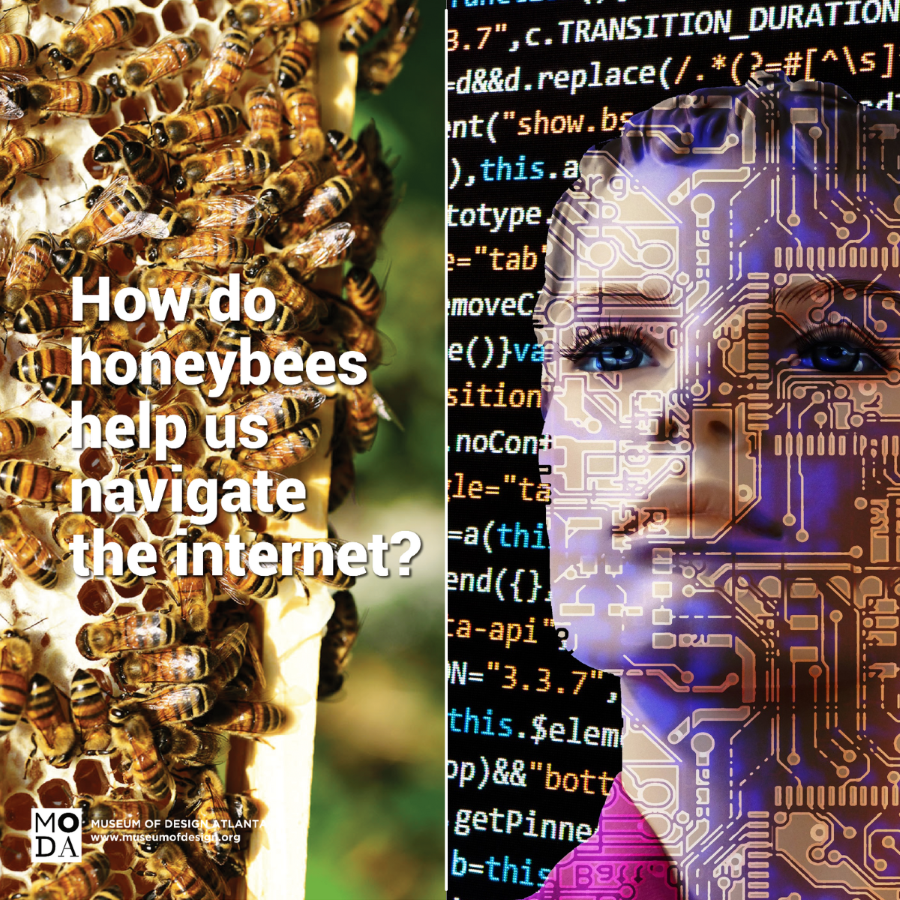
The Honeybee Algorithm
Things get complicated in the digital world when single servers host multiple websites for multiple clients. Decisions have to be made about how to allocate resources effectively so that websites with lots of traffic don’t use so many resources that other server clients suffer.
That’s a problem that Craig Tovey, a Professor in the College of Computing at Georgia Tech, and Sunil Nakrani, an Oxford University Ph.D. student, took on. Their inspiration? Honey bees!
During the course of his career, Tovey studied how honey bee colonies allocate their time and energy. In warm seasons, bee colonies must collect nectar in order to make and store the honey that will keep them alive in winter. Deciding how many bees to send to which flower patch is complicated, however. A large number of bees at one patch can exhaust the flowers’ capacity to generate nectar. And, some flower patches are richer or more productive than others. To maximize nectar intake, the honey bee colony must “decide” how many bees will forage at each flower patch.
Tovey was looking for an industrial application for his learning from bees when he met Nakrani, who was interested in finding a better way to allocate resources on servers. The two researchers began working together to develop an algorithm that mimics the behavior of honey bees, allowing servers to respond dynamically to constantly changing conditions.